New physics opportunities with heavy-ion collisions at the LHC
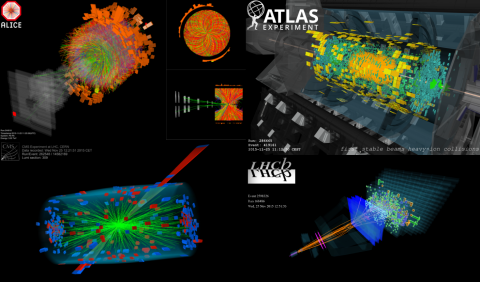
The LHC is the latest scientific tool in a long line of increasingly sophisticated machines built over the last 70 years to study the inner workings of our Universe and the laws governing its microscopic structure and evolution. Decades-long experimental and theoretical efforts have given rise to the Standard Model (SM) of particle physics; one of the most thoroughly tested scientific theories in the history of the human intellect. The SM currently provides our best understanding of the fundamental particles and their interactions. Despite the experimental absence of any definite signs of breakdown of the SM, a number of empirical facts and theoretical considerations remain unexplained within this framework, thereby calling for the need of an extended theory beyond the SM (BSM). The existence of dark matter, the neutrino masses, the matter-antimatter asymmetry, the lightness of the Higgs mass, the origin of fermion families and mixings, the early Universe inflation, and the cosmological constant are among the most fundamental questions that find no explanation within the SM today.
So far, the LHC experiments have collected and analysed about 5% of the total data that the LHC is expected to deliver by the end of its lifetime. Despite the successful discovery of the predicted Higgs boson in 2012, the absence of new particles or phenomena around the electroweak scale has triggered new ideas and novel experimental strategies by the LHC collaborations. Along with revisiting searches for more complicated supersymmetric scenarios, experimentalists are turning also their attention to new particles such as dak photons, right-handed neutrinos, axion-like particles, magnetic monopoles, and other classes of long-lived particles that could offer hints of new physics.
BSM physics may still be evading experimental observation either because it lies at higher energy scales (higher masses) or it couples feebly with the particles of the Standard Model. These options also define the strategy in designing future colliders that could offer direct reach at higher energies, and/or larger luminosities to search for weakly-coupled physics or very rare processes. At face value, both search strategies present obvious drawbacks for heavy-ion (HI) compared to proton collisions at the LHC, because the former are collected at comparatively lower collision energies and with orders-of-magnitude smaller luminosities. However, a new publication has recently summarised various unique opportunities that heavy-ion collisions present for BSM searches accessible during the High-Luminosity phase of the LHC after 2029. The diversity of these searches with heavy-ions beyond LHC Run 4 would offer unique access to the parameter space of some of these new physics phenomena, while complementing other approaches studied at colliders, in space, and at underground experiments.
A first reason that makes heavy-ion collisions attractive for BSM searches is the negligible pileup and improved vertexing compared to p-p collisions. As the authors explain: “Pileup translates into a rising difficulty to record all interesting p-p events, and thereby an unavoidable increase of the kinematical thresholds for triggers and reconstruction objects in order to reduce unwanted backgrounds. Pileup also leads to an intrinsic complication in the reconstruction of exclusive final-states and of displaced vertices from e.g. long-lived-particles (LLPs) that appear in many BSM scenarios.”. In addition to reduced trigger thresholds, heavy-ion collisions offer optimal primary vertexing thanks to the large number of primary tracks, as well as very large yields of exclusive final-states in photon-photon collisions through ultra-peripheral interactions. These facts provide new arguments to motivate the continuation of a heavy-ion programme beyond Run 4. The article makes the case that HI collisions can offer competitive access to a number of BSM searches using four underlying production mechanisms: photon-photon fusion in ultraperipheral collisions (UPCs), 'Schwinger' production through strong classical EM fields, hard scattering processes, and thermal production in the Quark-Gluon Plasma (QGP). Such searches provide additional motivations, beyond the traditional studies of the QGP properties and of the collective non-Abelian behaviour of quarks and gluons, to continue running with heavy-ions beyond Run-4.
The first of these mechanisms, two-photon fusion in UPCs, has low-mass cross sections enhanced by factors of Z4, namely of 45 million for lead ions, compared to p-p collisions. The highly relativistic ions provide very strong sources of electromagnetic (EM) radiation thanks to their large proton charge number Z, which are particularly useful for axion-like particle searches as well as for any BSM signals that couple to photons like radions and gravitons. This combination offers a natural environment for searching some of the above-mentioned BSM states that predominantly couple through QED interactions. These searches can also profit from both theoretical and detector development work that is ongoing for proton collisions at the LHC. Heavy-ion collisions will allow studying such photon-photon fusion processes in an environment free of pileup and without the need of forward proton tagging, allowing the probe of BSM diphoton objects with 5–100 GeV masses that are otherwise not accessible in p-p collisions.
Secondly, the Schwinger' production mode through strong classical EM fields presents a great opportunity for monopole discovery. Theoretically, the existence of magnetic monopoles easily explains the existence of charge quantisation in the SM. Such considerations have motivated their search, including the dedicated MoEDAL experiment at the LHC. Searches for monopoles produced in partonic scatterings in p-p collisions are limited by an enormous non-perturbative suppression that consequently limits their production cross-sections. These limitations do not apply when considering the classical QED production exploiting the large magnetic fields present in ultra-peripheral HI collisions. According to the authors of the paper: “Searches in HI collisions at the LHC could in principle produce 2–3 orders of magnitude heavier monopoles, directly testing their existence in the hundreds of GeV mass range for the first time.”
A third promising avenue is the exploration of BSM particles, mainly in the form of long-lived particles, in hard scattering processes. In most models, LLPs own their longevity to a comparably light mass, a feeble coupling to ordinary matter, or a combination of both. If such particles are produced in HI collisions, their feeble interaction allows most of them to leave the quark-gluon plasma unharmed. Due to their long lifetime, the tracks from their decay into SM particles can easily be distinguished from the large number of tracks that originate from the collision point given the absence of pileup in heavy-ion collisions. Competitive limits can thereby be extracted in the mass region of 1–10 GeV in ATLAS/CMS. In addition, searches for LLPs with heavy-ions could reveal new production mechanisms. These searches could complement current and future LLP searches with p-p collisions that profit from their much higher luminosity. In the case of low-mass dark photons A', the ALICE heavy-ion experiment proves also competitive thanks to its improved reconstruction and identification capabilities at low momenta compared to ATLAS and CMS. Most experimental searches focus on A' decays to dielectrons (if mA' < 2mμ), dimuons (for A' masses above twice the muon mass) or dihadrons, and have so far constrained its existence in the mixing parameter g2 versus mass mA’ plane. As an example of the HI feasibility, ALICE expects to reach a limit in g of about 10−4 at 90% confidence level (CL) during Run-3 for dark photons in the mass range of 20–90 MeV. Such limits may eventually be superseded by LHCb and fixed-target experiments, though an increase in the total HI integrated luminosities (e.g. running with lighter ions) can render them former competitive.
Finally, new BSM phenomena could arise due to thermal production in the QGP. Perhaps one of the most discussed examples is the sexaquark S state, a hypothetical stable dibaryon consisting of two up, two down, and two strange quarks that may account for the Dark Matter in the Universe. The mass of the hypothetical sexaquark is expected to be in the range mS ≈ 2mp ± mπ, and has escaped detection so far. Moreover, if a stable or weakly-decaying dibaryon exists, its production in heavy-ion collisions can be completely predicted as a function of its mass, and the temperature T and local baryon chemical potential μB of the produced QGP. Studies are ongoing to understand the accuracy by which different techniques can identify the production of S and Sbar, either exploiting the excellent hadron-identification capabilities over a wide momentum range in ALICE and LHCb, or the larger acceptance of the multi-purpose ATLAS and CMS detectors.
The unique physics opportunities described above would require extending the LHC heavy-ion runs beyond Run-4 and also the use of alternative lighter ion beams. In the present plan, it is foreseen to perform another 4.5 PbPb runs before the end of the LHC Run 4 in 2029, accumulating ~13 nb−1 (~100 pb-1 p-p equivalent) in total. Within the same period, one short pPb run, as well as one reference p-p run, are also planned. However, the full exploitation of the BSM possibilities opened up in heavy-ion collisions requires the largest possible integrated luminosities. A revised proposal for Runs 3 and 4, and plans to extend the LHC nuclear programme beyond Run 4 have been formulated –including more time for asymmetric pPb runs and collisions of lighter nuclei like Ar, O, or Kr– and is currently under review.
Note: You can read the full paper "New physics searches with heavy-ions at CERN's LHC" published on Journal of Physics G: Nuclear and Particle Physics (Vol. 47, 6, May 2020) here: